Some of the cosmic mysteries eluding explanation.
While it would seem that scientists and astronomers know an awful lot about the Universe, we are nowhere near knowing everything there is to know.
Yet every one in a while, astronomers make a breakthrough in our understanding of the Universe, and our perception of how the Universe works is substantially shifted.
Take for example the American astronomer Edwin Hubble, who in 1923 used the 100-inch Hooker Telescope at the Mount Wilson Observatory in California to photograph the Andromeda Galaxy.
Discover the biggest mysteries in the Universe and how close we are to understanding the Universe.
The calculations that resulted solved one of the biggest unanswered astronomical questions of the day.
Proving that Andromeda, and other ‘spiral nebulae’ like it were actually huge galaxies millions of lightyears away, rather than small objects closer by, within the Milky Way.
The discovery changed astronomy forever by showing just how vast our Universe truly is.
Technology may have massively improved since then, but there are still many unsolved mysteries surrounding our Universe.
Here are some of the most pressing issues occupying the finest astronomical minds of the 21st century.
What we still don’t know about the Universe
How supermassive black holes merge

When a black hole smashes into another black hole, it sends shock waves rippling out through space itself: gravitational waves.
Yet so far the mergers we’ve seen have come from so-called stellar-mass black holes – those with masses a few tens of times the mass of our Sun.
That’s tiny compared to a supermassive black hole, which can tip the scales at billions of solar masses.
There’s a supermassive black hole in the centre of every major galaxy and galaxies also collide, so what happens to the two black holes at their hearts?
You’d think that they’d merge too, but there’s a problem.

To spiral inwards, the black holes need to lose energy, which they do by shedding heat to surrounding gas, dust and stars.
Yet when they get within a parsec of each other – about 3.26 lightyears – there’s no longer enough stuff to lose energy to.
This ‘final parsec problem’ means the supermassive black holes should remain orbiting each other and never actually merge.
However, recent evidence from the NANOGrav experiment suggests that the Universe is humming with gravitational waves from supermassive black hole mergers.
The data from this hum might give astronomers the clues they need to finally unpick this problem.
What the Universe actually looks like

Look at the large-scale structure of the Universe today and countless galaxies are strung out on endless filaments in a cosmic web.
It seems that tiny variations in density in the very early Universe acted as seeds from which this structure eventually blossomed.
Over-dense regions drew in more and more material to create galaxy-filled superclusters and leave behind supervoids.
Except the Big Bang theory says that the early Universe should have been completely smooth.
How come it had tiny imperfections of just a few parts in one hundred thousand, but which created the Universe as we see it today?

One explanation is a modification to the Big Bang, called inflation, which states what happened in the first few seconds of the Universe.
It argues that the Universe underwent a period of super-rapid expansion in the first miniscule fraction of a second.
The cosmos went from considerably smaller than an atom to about the size of a grapefruit in a trillionth of a trillionth of a trillionth of a second.
Before this, the Universe was so small that it was dominated by minute quantum variations.
The ensuing explosion in size blew up those variations.
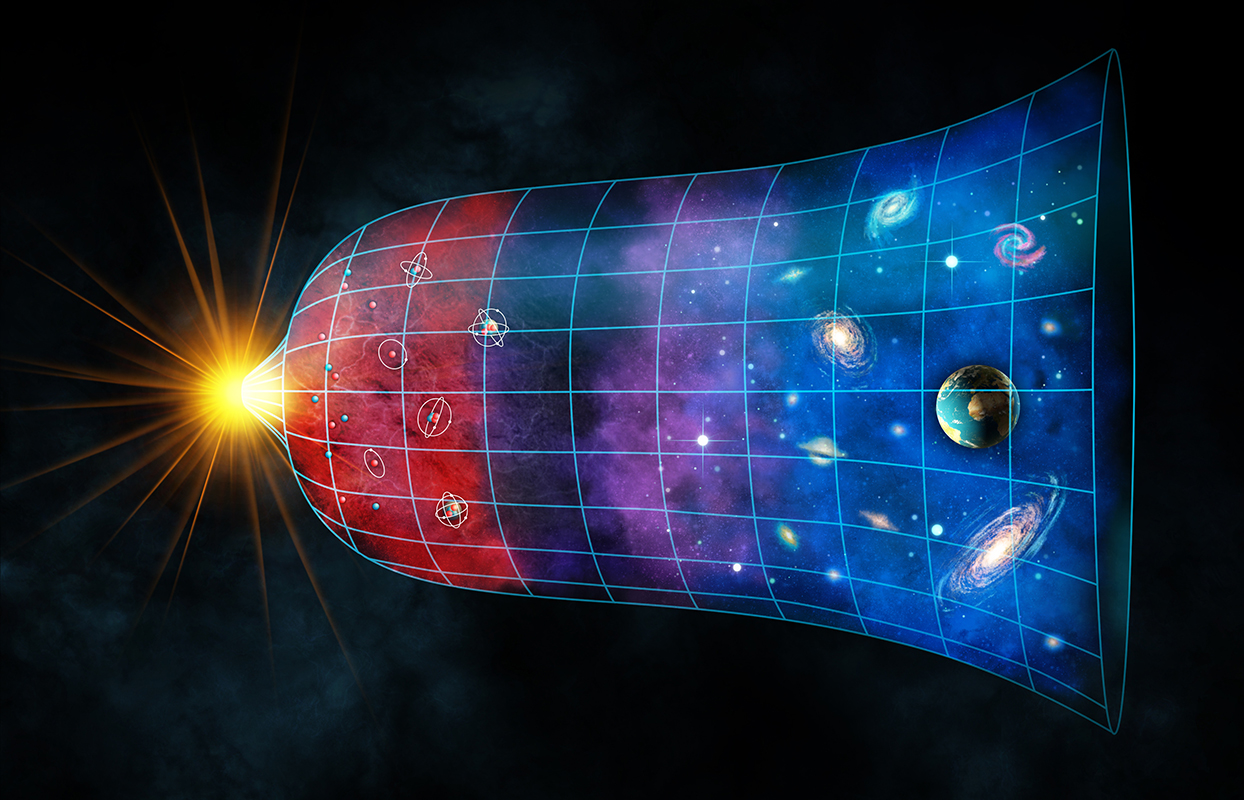
It’s worth saying that currently there’s no evidence that inflation really happened, although it does help solve other problems with the Big Bang too.
Nor do we know the Universe’s true size.
Astronomers speak about the observable Universe – a spherical region 13.8 billion lightyears in all directions where light has had time to reach us since the Big Bang.
However, there could well be things beyond this cosmic horizon that are so far away the light hasn’t arrived at Earth yet.
This also makes it difficult to determine what shape the Universe is.
Astronomers currently believe the 4D fabric of space-time is flat, but it could simply be that inflation has smoothed out the billions of lightyears we can see, and beyond that the Universe is actually curved.
One thing we don’t know about the Universe it its shape.
What gravity really is

While we know what gravity does, it’s unclear how it does it.
Our current best theory of gravity is Einstein’s general theory of relativity.
It says that massive objects like the Sun warp space-time, the fabric of the Universe, around them.
Earth is then caught in the distortion that the Sun makes, which is why we orbit.
Except this isn’t how physicists treat any of the three other fundamental forces. They are all carried by particles called bosons.
Magnets attract each other, for example, by exchanging bosons called photons.
So the hunt is on for the graviton – a so-far-hypothetical particle that would be responsible for gravity.
What happens inside a black hole

One of the most asked questions about black holes is “what would happen if you fell into a black hole?”
Wander too close to the horizon of a black hole and the extreme difference in gravity between your head and your feet would rip you apart into lengths of human spaghetti.
But where does that pasta eventually end up?
Our best theory of black holes is Einstein’s general theory of relativity.
It says that inside a black hole space becomes increasingly concentrated into an ever-smaller volume.
In fact, at the very bottom space is crushed into an infinitely small speck called a singularity.
However, a singularity is likely to be a placeholder for our ignorance.
The curvature of space becomes infinite and the singularity’s density becomes undefined.
In other words, the laws of physics suddenly lose their predictive powers.
The elephant in the room is quantum physics.
If you squeeze space down into a volume smaller than an atom, then quantum physics probably has something to say on what happens.
However, despite many valiant attempts, physicists have yet to combine general relativity and quantum physics in a satisfactory way.
If we one day create a so-called Theory of Everything, we may find out what we need to replace a singularity with.
What dark matter is

There’s something about galaxies that just doesn’t make sense – they’re rotating far too fast.
So fast that their outer stars should fly off into space, but that’s not happening.
Instead, astronomers suspect that galaxies contain an invisible gravitational glue called dark matter that helps to keep hold of those stars.
But what is dark matter made of? This is one of the most significant things we don’t know about the Universe, in that it’s a key unanswered question.
For decades the leading idea has been something called Weakly Interacting Massive Particles (WIMPs).
Except physicists have gone to great lengths and performed extensive and exhaustive searches for them, and they haven’t seen a single one.
And so they’re starting to look seriously at other options.

Other candidates include that dark matter is caused by the gravity of tiny black holes formed shortly after the Big Bang, known as primordial black holes.
Or dark matter could be particles called axions, which physicists conjured up to solve a problem in particle physics.
There’s a chance that dark matter isn’t actually a physical substance at all, but an illusion instead.
This is the premise behind a theory known as Modified Newtonian Dynamics (MOND).
It says that the ‘missing gravity’ is actually due to the fact that gravity works differently on the scale of galaxies and we’ve been misinterpreting the situation.
Which way up galaxies form

There are hundreds of billions – perhaps even trillions – of galaxies in the modern Universe, but where did all these star-cities come from?
There appear to be two ways to make a galaxy.
You could start small with individual stars and minor star clusters.
Over time, gravity, added by the glue of dark matter, could then bind lots of these together to form the conglomerations we call galaxies.
This is a so-called ‘bottom-up’ approach.
The alternative is ‘top-down’, where huge structures form early in the Universe’s history only to fragment into galaxies as the cosmos aged.
The James Webb Space Telescope is currently wowing astronomers with insights into the early Universe.

It has seen fully-fledged galaxies just 400 million years after the Big Bang and even some that appear to be merging.
So that seems to favour the bottom-up approach, but it needn’t be a binary situation. It may be possible for some galaxies to form bottom-up, while others formed top-down.
Huge upcoming galaxy surveys like those with the Vera Rubin Observatory in Chile could help us to know more.
Plus the Webb Telescope is only just getting started.
How fast the Universe is expanding

When Edwin Hubble discovered cosmic expansion in the 1920s, it was a major clue that the Universe began with a Big Bang – the point at which the expansion began.
Hubble found that distant galaxies appear to be moving away from us faster than those nearer to us.
Galaxies aren’t really moving through space, though – it’s the space between galaxies that’s stretching as the Universe expands.
The more space there was between us and a galaxy to begin with, the faster that galaxy seems to recede when that space gets bigger.
This led to the idea of a so-called Hubble constant, which measures the rate of expansion in the current Universe.

When measured from objects in the nearby cosmos, it has a value of 73 kilometres per second per megaparsec (km/s/Mpc).
That means a galaxy a million parsecs away (3.26 million lightyears) appears to be receding at 73 kilometres per second.
A galaxy twice as far away seems to be fleeing from us twice as fast.
But there is another way astronomers can measure the Hubble constant: using the Cosmic Microwave Background (CMB).
The CMB is the relic radiation left over from the Big Bang, but it can be used to calculate the current constant.

However, when you do this the answer you get is 68 kilometres per second per megaparsec – about 7.5% less than the value obtained from fleeing galaxies.
This discrepancy has become known as the Hubble tension.
While both measurements have been verified multiple times using several different methods, it could be that one of the measurements is somehow wrong.
There are also ways that both could be right – for instance, the local measurements could be skewed if we are in an unusual part of the Universe, or there could be some underlying physics we don’t know about that’s waiting to be discovered.
Why there’s more matter than antimatter

One key thing we don’t know about the universe is how it actually exists at all, and this relates to a phenomena known as antimatter.
Every subatomic particle has a mirror image – a particle of antimatter.
When an ordinary particle meets its antimatter counterpart they annihilate in a flash of light.
Matter and antimatter should have been created in equal quantities after the Big Bang and should have completely annihilated by now.
Yet the Universe is full of matter and very little antimatter.
Astronomers suspect that for every billion antimatter particles, a billion and one matter particles appeared.
All the antimatter annihilated with almost all the matter, leaving a tiny residue of matter.
Yet we still don’t know why nature has a slight preference for matter over antimatter.
How the Universe will end

Astronomers used to think that the gravity of everything in the Universe would eventually pull the cosmos back in on itself in a reversal of the Big Bang, dubbed the Big Crunch.
However, that was before they discovered that the expansion of the Universe is accelerating due to a shadowy entity known as dark energy.
If it continues to accelerate, all structures in the Universe – even atoms – will be torn apart within 22 billion years in a so-called Big Rip.
A more sedate expansion would lead to a Big Freeze instead, where the expansion of the Universe causes it to cool so much that stars cannot shine and the Universe eventually settles into a uniform temperature.
Find out more about this in our interview with Katie Mack.
Do you have any questions about the Universe you’d like answered? Get in touch by emailing contactus@skyatnightmagazine.com
This article originally appeared in the October 2023 issue of BBC Sky at Night Magazine