The science author discusses his new book, A Crack In Everything.
It’s been roughly 100 years since the idea of a black hole was first mathematically predicted, and since that time science has witnessed their confirmed detection and even the capture of the first images of these incredible objects.
Black holes have gone from relative obscurity to becoming key to our understanding of general relativity, spacetime, galaxy evolution and the expansion of the Universe.
And yet, we still understand so little about them; we still have so much to learn.
This is the central premise of A Crack in Everything, the latest book by former radio astronomer and science author Marcus Chown.
We got the chance to speak to Marcus about why black holes are so important, and why understanding them fully will enable us to solve some of the biggest mysteries in the Universe.
What is the ‘crack in everything’?
Basically, the major theories of physics, which are general relativity (a theory of big things like stars and galaxies), a theory of small things, quantum theory (atoms and those kind of things), thermodynamics (a theory of heat), all collide in black holes.
They all predict different things for the same situation. And for physicists that’s gold dust, because it means that one or more of those theories is incorrect.
So really, the crack is the cracking in physics itself.
You can think of science as accumulated knowledge, but reading the book, it feels like there are specific moments and individuals that propel everything forward. Is that a more accurate depiction of science?
I’m fascinated by the way science is done, because it’s actually kind of like a drunkard’s walk, and there are often people reaching dead ends, getting the wrong answer, or getting the right answer for the wrong reason.
George Gamow came up with the idea of the Big Bang, but he thought there was an oscillating universe, so he got the right answer for the wrong reason.
But with black holes, there are a number of individuals who are really important, and interestingly my editor said to me that towards the end of the book I don’t seem to be talking about individuals any more.
But because this topic spans the last hundred years, there’s been a fundamental shift in physics, from individuals being able to make huge contributions to only giant teams.
So actually the book documents that. It’s easy at the beginning of the story because there are people like Karl Schwarzschild, Einstein, Subrahmanyan Chandrasekhar, these kind of individuals.
Roy Kerr was the New Zealander who found the description of spacetime around a spinning black hole.
These are individuals, but then you move to these great teams of people like the Event Horizon Telescope, large team of people who got the first ever image of a black hole.

As a writer you’re trying to impose a kind of logic on it, and of course this is not the way it was actually experienced by the people involved.
Certainly the first part of the 20th century, people didn’t think that black holes were serious.
And they thought that if they existed, they would be black, small and invisible and so there would be no point in searching for them.
But we, looking back with hindsight, can impose a structure on this history, and that’s what I’ve tried to do.
The book opens with Karl Schwarzschild in his hospital bed in the First World War, and he comes up with the idea of a black hole from there. Not from having observed anything or run simulations through a computer. He comes up with a black hole using mathematics and a piece of paper. How is that possible?
Well, it’s interesting because my previous book was called The Magicians, and it was precisely about that.
It’s about how in modern science, discoveries are made by someone scrolling symbols on a piece of paper.
It starts with Neptune in the 19th century, when Urban Le Verrier predicts the existence of this planet and tells people exactly where to find it.
To me that’s the central magic of science, that you can scroll these symbols down on a piece of paper and they can predict things in the real world that no one has ever suspected, and we go and find them.
Things like the Higgs particle, things like black holes.
This is an incredible miracle because it means there are two universes.
There’s the universe that we live in and there’s a mathematical universe that you can write down on a piece of paper and it is an exact analog of the real Universe.
And nobody knows why that’s true.
Paul Dirac, the famous British quantum physicist, said “God must be a mathematician”.
We don’t actually know why the universe has this complete parallel, this complete analogue that you can write down on a piece of paper.

One of the points you make in the book is black holes are neither black, nor holes!
‘Black hole’ is a fantastic name because in the 20th century there was very little interest or investigation of black holes, theoretically, and very few thought they could actually be detected.
In 1967, John Wheeler, who was the supervisor of Richard Feynman, came up with the name ‘black hole’.
In fact, Wheeler always said it wasn’t he who came up with the name.
He kept talking about these warpages of spacetime and then somebody in his audience, a student, said “why don’t you just call them black holes?”
So he then introduced the term. And suddenly you get this explosion of interest because there’s a term that paints a vivid picture in people’s minds.
We now talk about ‘losing something down a black hole’. So it’s entered the language and there’s pretty much nobody on the planet who doesn’t know the phrase black hole.
But one of the central characteristics of the black holes we’ve discovered is they are blazingly bright.
In fact, black holes are some of the most luminous objects in the entire Universe.
And far from us seeing material sucked in, one of the central characteristics is that material is coming out of black holes.
So we see the supermassive black holes in the centre of galaxies with these incredible jets of material that are directed from the poles of spinning black holes and extend across millions of lightyears.

The term ‘black hole’ does, I suppose, give you the idea that material falls in and never comes out.
But they’re not black at all. And that really did surprise people. And it shouldn’t have surprised people.
Stuff is not going to fall directly into a black hole, because it’s got angular momentum, because it’s spinning.
It will swirl around like water, running down the plug hole.
And we now know that friction within that gas will heat it to millions of degrees.
What we see is not directly the black hole, but the blisteringly hot accretion disk around black holes, which make them so phenomenally luminous.

When we see an image of a spiral galaxy, is that bright centre a result of the supermassive black hole at the core?
First of all, we thought black holes must be the preserve of science fiction, then we begin to think that maybe they’re not.
Then we discover them, and then we discover that they power some of the most powerful galaxies in the Universe.
About 1% of galaxies are active galaxies. That means most of their light does not come from stars, but an accretion disk around a supermassive black hole.
This is a black hole that’s maybe millions to 10s of billions of times the mass of the Sun.
And that makes these objects phenomenally bright, and the most famous example would be quasars.
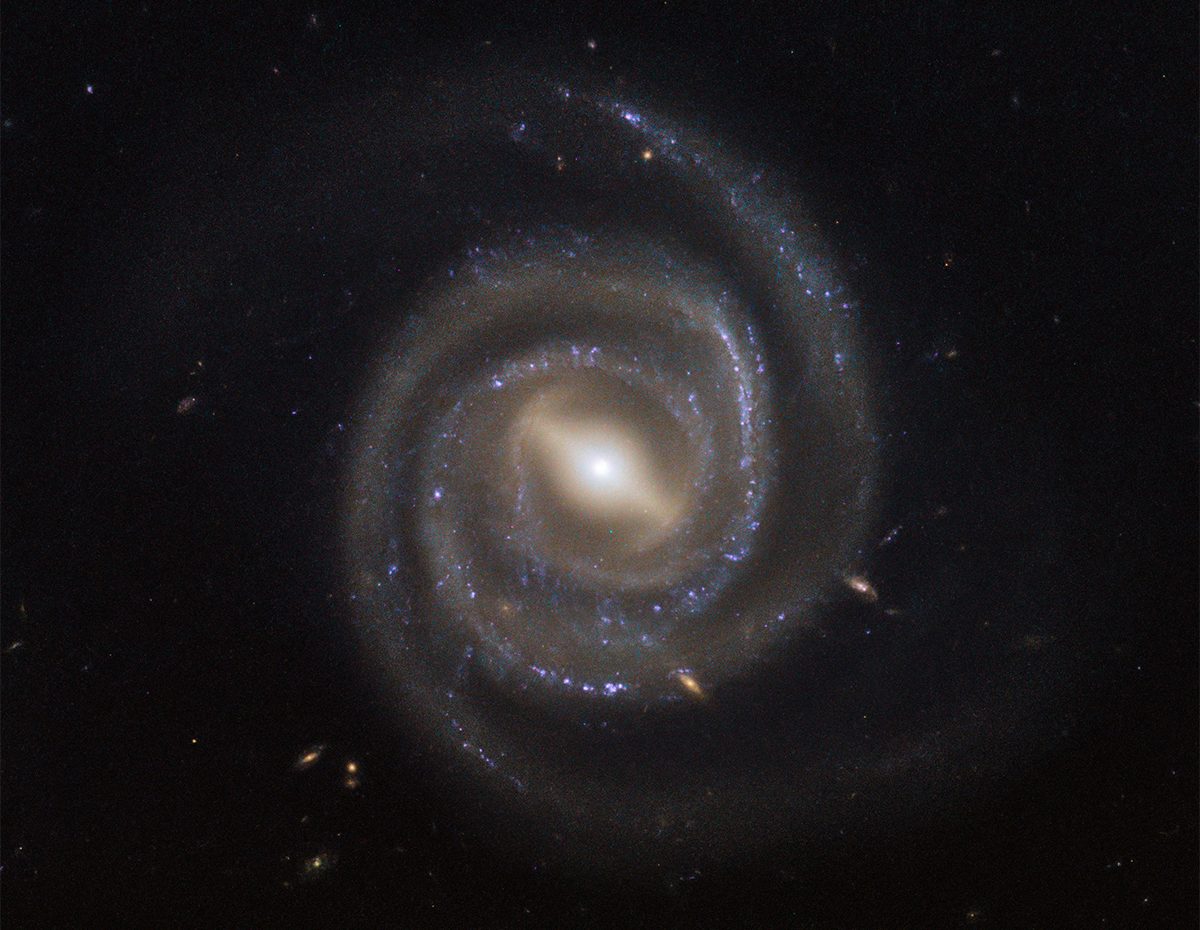
Their nuclei are so bright that it took a long time, probably 20 years, before our telescopes, our detectors, were good enough to detect the stars surrounding them.
This is like trying to detect a few fireflies when there’s a whopping great searchlight in your eyes.
These accretion disks pump out about a hundred times maybe even a thousand times the energy of the stars and completely wipe them out.
Even when we discovered them in galaxies, we thought they were still anomalous, not important. They must be peripheral objects to the Universe.
Then we discover in fact they’re in every single galaxy, and the reason we only see them in 1% is because in 99% of galaxies they’re slumbering.
They’re not active, they’ve run out of food.
This was a major discovery because we thought they were these incredible anomalies, of no significance to the Universe at all, then we find that they’re in 1% of galaxies, powering things like quasars.
And we can still say, well, they’re not very significant. That’s only 1%.

But then in the 1990s, because the Hubble Space Telescope was placed above Earth’s distorting atmosphere, it had very sharp vision and could look into the centres of many nearby galaxies.
And in every single one, the stars were whirling around far too fast.
Therefore, they were in the grip of some kind of concentrated mass.
So during the 1990s, the Hubble Space Telescope discovered that there was actually a supermassive black hole in every galaxy.
That’s one of the great mysteries. Why is there a supermassive black hole in the centre of every galaxy, including our own?
Which forms first, the supermassive black hole or the galaxy?
We’ve got no idea. We don’t know. We really don’t know. We are hoping that the James Webb Space Telescope will shed some light on this.
It can look back to very early times. It’s seen quite large supermassive black holes very early on in the Universe.
We don’t know whether maybe a gas cloud collapses to form a supermassive black hole and then the galaxy accretes around it, or whether the stars form first and then form a cluster at the centre, they begin to collide, form black holes, which merge to become supermassive black holes.
This is, again, a huge problem for astrophysics because we see evidence of these supermassive black holes extremely early on, within the first few hundred million years, maybe two or three hundred million years after the Big Bang.
And it’s very hard to see how they could grow that quickly.

So there are two problems.
To get a big black hole, you need a reasonable seed to start with, and then it needs to be able to accrete material.
And as far as we know, we think the first generation of stars to form after the Big Bang, the first stars to form in the very first galaxies, were a bit more massive than the ones that we see now.
But there’s a reason for believing they were more massive
They were called Population 3 stars, and they would have left relatively big black holes, maybe 20, 30 times the mass of the Sun.
But that doesn’t seem to be big enough to end up with something that weighs almost a billion solar masses, within maybe 500 or 700 million years of the Big Bang
The big problem is that as they accrete material, the accretion disks become extremely hot and the radiation pouring outwards pushes away any incoming materials.
That is what we call an Eddington limit to how fast a black hole can grow.
So to get some of the biggest ones that we’re seeing in the early Universe, you need very large seeds and you need them growing at absolutely the maximum rate possible.
So, again, the whole point of my book is that these objects have become central to galaxies and our being here.
But the big questions about how, why, where they came from, what they’re doing in the centre of all galaxies, how they got so big so quickly, these things are not known.

There’s a lot of discussion that Webb is seeing things in the early Universe that shouldn’t exist. Does it give you hope that a powerful observatory like Webb will be able to solve mysteries like that?
Definitely. It’s gold dust to physics. You started off by asking me about why black holes are a crack in everything, and it’s because they show us where physics breaks down.
And that’s a great help to finding a better, deeper theory of physics than we actually currently have.
It’s very exciting when we know that there’s something big that’s missing, that we don’t understand.
One of the most bizarre things about supermassive black holes is they are incredibly tiny.
A supermassive black hole is pretty much like a bacterium compared to London.
You would expect that it would have no effect on its galaxy, and in terms of mass, generally they are about a two hundredth the mass of even the central bulge of stars, so no one expected they would have any effect whatsoever on their large scale galaxy.
Their gravitational influence shouldn’t extend more than 10 or 20 lightyears when the central areas of galaxies are maybe 20,000 light years across.
And yet, it was discovered again, since the year 2000, they have left an indelible imprint on the stars in their galaxy.
In pretty much every galaxy we see, the stars are about 200 times the mass of the supermassive black hole.
They’ve obviously grown together and influenced each other.
They can’t today, it’s impossible, but they must have done very early in the galaxy’s history.
I think the key is not size, size does not matter, it’s actually the energy.

If you walk past a house and a slate falls on your head. It hurts. And that’s because of what we call gravitational potential energy.
That’s the energy that the slate had by virtue of its position in the gravitational field, was converted into kinetic energy and hit you on the head.
When you form a supermassive black hole billions of times the mass of the Sun, it’s like God knows how many quadrillion slates all coming together.
And the energy required to do that is about a thousand times more than the energy necessary to actually explode the galaxy, in other words blow apart all of the stars to infinity.
So there’s more than enough energy there for the formation process of a supermassive black hole to leave its imprint on the gas which later forms the stars and leave some kind of imprint.
Until it was realised that this imprint had been left, we were missing a major ingredient in our understanding of how galaxies form.
Galaxies make no sense whatsoever if you don’t know about supermassive black holes.
They obviously play some key role in the formation of the stars early on in galactic history.

It’s incredible that black holes were ever ignored and dismissed!
It’s as if physicists and astrophysicists have been dragged kicking and screaming to this realisation that they play an enormous role in our Universe.
And in fact, we’re only having this conversation because of the supermassive black hole in the centre of our galaxy. Sagittarius A* is a tiddler. We’ve got no idea why it’s so small.
We should have a black hole of about maybe half a billion solar masses in the centre of our galaxy.
It’s about a thousand times smaller than you’d expect for a galaxy of our size.
If you think about what it would be like in the galaxies with the really big supermassive black holes, which have these incredible accretion disks that glow at millions of degrees.
There’s these huge outflows of material coming from them, heat and matter driving away the raw material that would make stars.
So in these really big galaxies, with the big supermassive black holes, we see only one generation of stars.
Very early on, stars formed and then all the gas got blown away.
And those stars would have been stars made from the pristine material from the Big Bang, so they would only have had hydrogen and helium, they wouldn’t have had the heavy atoms like calcium and neon etc.
And all of those things like calcium and carbon are necessary for life.
So the big galaxies with the big supermassive black holes would be biological wastelands.
There would be no planets made from heavy elements like the Earth. There would be no biology.
So here we find ourselves in this galaxy with a very tiny supermassive black hole where none of the gas has been blown away, and we’ve had multiple generations of stars.
Our Sun is believed to be a third generation star, so the first generation cooked some heavy elements.
They were splurged into space when those stars exploded, they became the raw material of the next generation.
We live in a galaxy contaminated with the elements of life. There’s no way we could have arisen in a galaxy with a very large supermassive black hole.

Does that raise problems if you try and look at the probability for life existing in our galaxy and extrapolate that out into the wider Universe?
Probably, yeah. We increasingly find other things, like we’ve got a Moon, as you know, which is very big, probably the biggest Moon compared to its planet.
Apart from Pluto, but then it’s a dwarf planet and has a moon called Charon.
We think the Moon played a big part in us being here, because it stabilised the Earth’s spin.
So the climate has remained pretty stable over billions of years necessary for the evolution of life.
We’re in this unusual galaxy with a very small supermassive black hole for its size.
We have a very large Moon.
All of these things certainly make it seem life as we know it might not be very common, but maybe there’s life as we don’t know it! Who knows?

Are black holes the reason you got into science?
When I was at school, they never mentioned astronomy.
But I was very lucky to be a nine year old when the first human being stepped on another world.
There’s a shiver going down my spine even telling you about that.
My dad got me up to see these fuzzy images and it just blew my mind.
My dad had left school when he was 15. He’d grown up during the war, they had no schooling, bought me a book on astronomy when I was about about eight or nine.
It was called Dr. H. C. King’s. Book of Astronomy. The author was the director of the London Planetarium, and I just got really into it.
I had a small telescope, which I poked out of the window of our council flat in North London.
I remember seeing Jupiter’s moons above the orange glow of the North Circular Road.
I was a member of the Junior Astronomical Society, and my dad took me to a meeting in Victoria in London, and a man on crutches hobbled towards the stage.
I didn’t realise that it was because he’d had polio, and his name was Paul Murdin.
He talked about Cygnus X1, which was the first black hole to be discovered, and it just blew my mind.
I got to interview him, and I don’t think people remember who he is.
There have been three Nobel Prizes for black holes now. No one ever mentions who discovered them, and in particular, the co-discoverer Louise Webster.
So if you really want to say what my book is about, it’s about people.
I want Louise Webster to get the credit that she deserves, because she was the co-discoverer of black holes.
She’s been basically forgotten, written out of the history of science.
If you think about it, the two endpoints of the lives of massive stars, what we call relativistic stars, the two types of stars that can only be understood using Einstein’s theory of gravity, they are pulsars, or neutron stars, and black holes, and both discovered by women.
Three Nobel Prizes have gone for pulsars, and not one has gone to Jocelyn Bell, who discovered pulsars, and Louise Webster’s in a worse position. She’s been entirely forgotten.

Fast forward to 2019 and that first image of the black hole. What did that mean to you?
I just think it was astonishing. It’s one of the key images in the history of science.
To see that image of a black hole was absolutely incredible because inside a black hole, we believe that space and time actually come apart.
We don’t actually have a theory that describes what happens, but we think they just come apart.
I think we have a tendency to beat ourselves up and think, we’re human beings, we’ve overpopulated the planet, we triggered global warming, pollution, all these kind of things.
But I think sometimes we need to pat ourselves on the back and think, we came down from the trees three million years ago, we have a three-pound brain made of jelly and water, but we have seen to the edge of space and time.
And I think that’s an incredible thing.